The additive manufacturing industry is growing at a compound annual growth rate of 24.3% [1] and by 2030 there will be over 2.7 million 3D printing devices in the world [2]. Alongside the medical sector, aerospace and automotive are amongst the top industries exploiting this rapid rise in additive manufacturing capability.
As these industries continue to find new applications for 3D printing technology, the physical properties of printing materials are being pushed to their limits. Consequently, companies like Conflux are undertaking major R&D projects to discover new materials and develop techniques that can unlock performance from metallic printing powders.
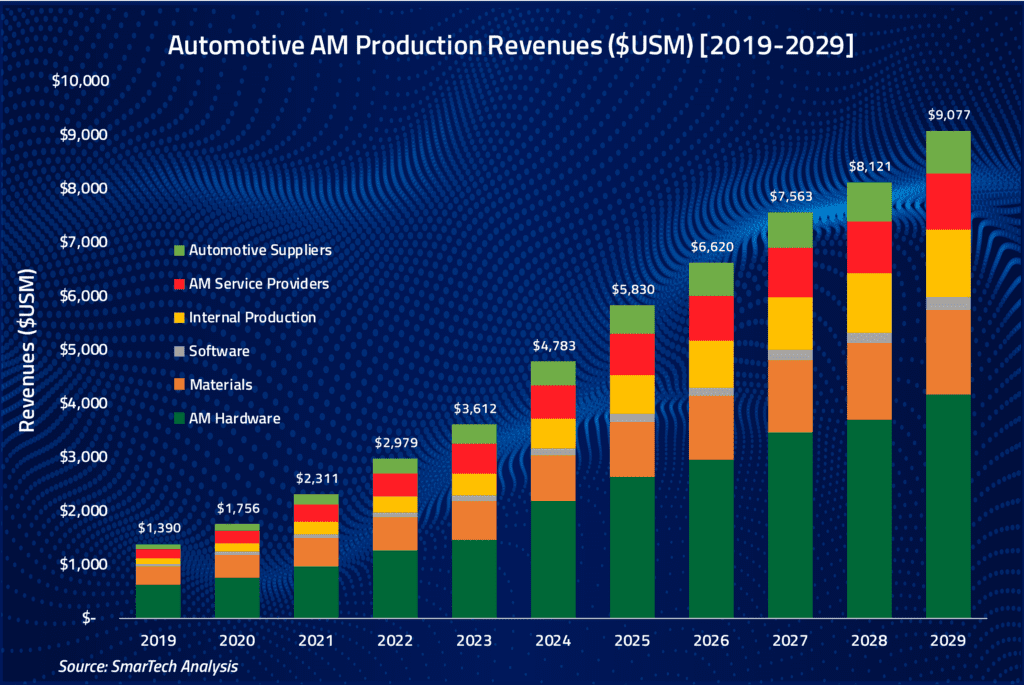
How does 3D metal printing work?
There are several methods to 3D print metallic components, all of which are based on the same basic principles where a CAD model is used as a template to build up a physical component in thin layers. Laser Powder Bed Fusion (LPBF) is one of the most widely used techniques which utilises a laser to fuse layers of metallic powder. It is a popular method for many practical reasons, such as ease of handling and recycling of the raw materials, but for Conflux the benefits lie in the accuracy of the component builds.
To achieve accurate builds with high heat transfer properties, choosing the most suitable printing powder is of paramount importance. There are a variety of metallic powders available for 3D printing, but not all can cope with the intense environment of a heat exchanger.
‘For high performance heat exchangers, we are always trying to make the walls and fins as thin as possible,’ highlights Dr. Ian Fordyce, Additive Manufacturing Engineer at Conflux. ‘The thicknesses we are dealing with are of the same order of magnitude as the particle diameters of the powder and the laser spot size itself. So, an error as small as 10 microns is a massive deal for us. This could cut the number of fins we can put in the heat exchanger as well as affect the surface roughness and increase wall thickness, all reducing the thermal performance of our heat exchangers.’
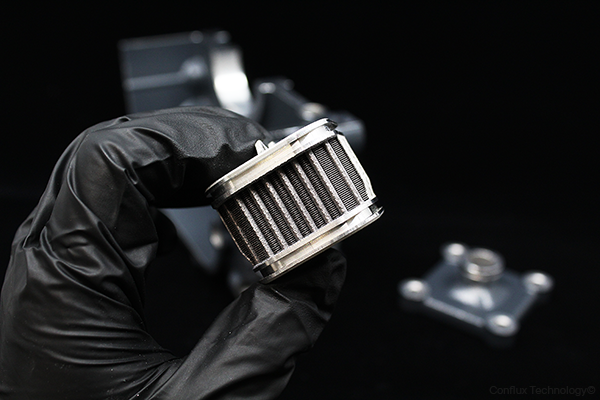
The characteristics of high-performance metal printing powders
The first stage to selecting a material is understanding its printability, which can vary hugely between powders. Printability is defined as the ability of a material to physically print geometries without causing the build to fail. Hard steels and nickel alloys for example, experience residual stress and warping during printing which causes them to lift off the build plate and crash into the re-coater. While other materials will print with low density, resulting in holes that can lead to failures.
The key to achieving high quality builds is to use powders with consistent particle size distribution and optimum composition. To guarantee this, Conflux follow strict quality control procedures where they track the composition of new and recycled powder using spectrometry analysis and mechanical testing of tensile bars. This not only monitors the levels of each alloy within a powder, but also the amount of oxide layers built up during recycling, which can then be accounted for with printing parameters.
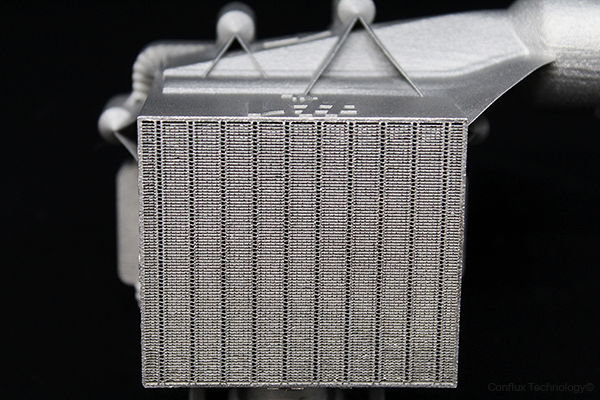
Once the powder has been deemed printable, the focus shifts to analysing the physical properties of the printed material. The alloys used for some heat exchangers need to cope with temperatures above 800°C. Alongside this, they also need to have a high fatigue life and corrosion resistance due to the variety of coolants used in automotive, aerospace and energy applications.
‘When it comes to thermal performance, the most important property for us is strength,’ explains Fordyce. ‘If we can use a higher strength material, then we can have thinner walls which shortens the path for heat transfer and ultimately means a higher performing heat exchanger.’
‘Most people assume that thermal conductivity is also important, but we’ve found it becomes less significant at the wall thicknesses we are dealing with. Copper, for example, has higher thermal conductivity than aluminium, but if we have to make the walls thicker to make the copper part strong enough, that results in a worse preforming heat exchanger overall. So, we can sacrifice thermal conductivity if it means we can achieve a part with thinner walls.’
Types of metal printing powders used in additive manufacturing
Stainless Steel Alloys
One of the materials in Conflux’s repertoire is 316 stainless steel which is a relatively stable alloy suitable for both high and low temperature harsh environments that require high corrosion resistance. This makes it perfect for manufacturing tools and machinery components for the oil and gas industry as well as engine components that come into contact with corrosive substances.
However, it is relatively heavy and therefore not suited for lightweight applications in aerospace or motorsport. It can also experience higher warping and residual stresses during printing then aluminium.
Titanium Alloys
An extremely strong and lightweight material ideal for aerospace and motorsport applications is titanium, particularly titanium alloys such as Ti64. Since 2017, Airbus have fitted 3D printed titanium shafts to the doors of A350 airliners and recently opened an Additive Manufacturing Centre in Germany to produce parts for helicopters and lightweight Urban Air Vehicles (UAV) [3]. While IndyCar utilise 3D printed titanium for the frame that supports its aeroscreen driver protection device. It also exhibits high corrosion resistance, which can be further enhanced with oxide coatings.
Copper Alloys
The high thermal conductivity of copper makes it ideal for thermal applications such as heat sinks and heat exchangers as well as electronic components like busbars and induction coils. Unfortunately, it can be difficult to print with the type of laser wavelengths used in most additive manufacturing printers. Material manufacturers have recognised this and are now adding alloy elements to create high percentage copper alloys such as copper zirconium and copper chromium. These are much more printable and retain high thermal conductivity.
Aluminium Alloys
Aluminium alloys such as AlSi10Mg are the most commonly used material in 3D printing. This is mainly because aluminium solidifies with lower tendency to residual stress cracking compared to other alloys, which is why it has been used in casting for decades.
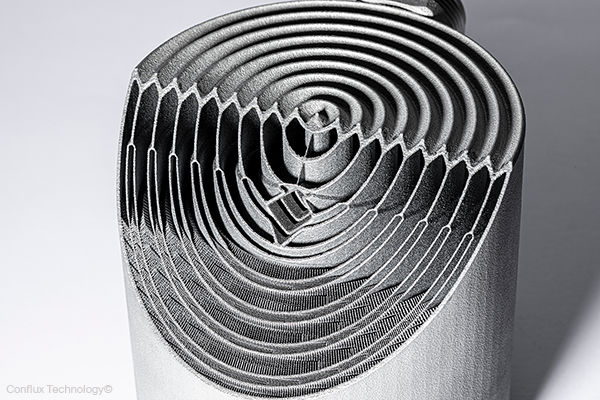
It is a cheaper alternative to titanium and so often used in aerospace, automotive and motorsport. Companies such as Airbus and Boeing continue to manufacture 3D printed aluminium components for its aircraft, helicopter and satellites – with the latter often featuring more than 500 additively manufactured parts. Aluminium can also be used to print Formula 1 heat exchangers and automotive pistons such as for Porsche’s 911 GT2 RS model.
Recently, there has been a lot of development to improve the printability of aluminium alloys that have a higher strength and corrosion resistance than AlSi10Mg. This has led to a variety of new powders that weren’t on the market a few years ago.
Nickel Alloys
Nickel alloys such as Inconel and Monel K500 maintain their strength at extreme temperatures and are resistant to highly corrosive fluids, making them perfect for rocket applications, gas turbine engine parts and other aerospace turbomachinery. However, printability can be an issue, as nickel alloys tend to develop residual stress and cracking during the printing process. These risks of build failures make this material expensive to print and so is typically only used for very specific applications.
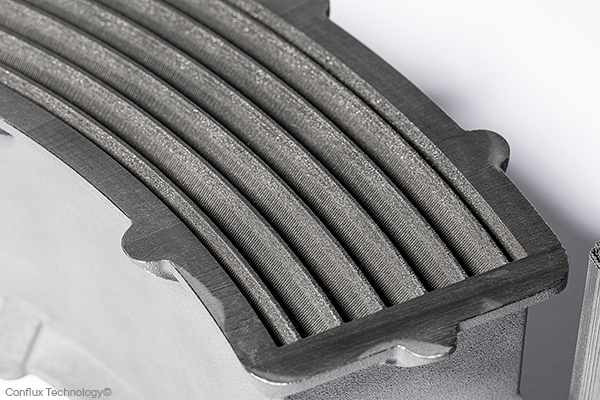
How coatings like Plasma Electrolytic Oxidation (PEO) can enhance performance of 3D printed parts
Another tactic to improving the performance of 3D printed components is to apply a protective coating on the part’s surface. This is particularly important to boost the corrosion resistance of aluminium.
‘There are several different methods of coating 3D printed parts,’ explains Dr Milad Laghaei, Associate Research Fellow at Deakin University. ‘The most common is painting, but for parts with complex geometries like Conflux’s heat exchangers, we cannot coat internal surfaces fully and risk blocking the intricate channels, compromising heat transfer.’
Additionally, paint may not withstand high temperatures or offer necessary chemical resistance, leading to degradation, potential contamination, and compromised performance.
An alternative electrochemical coating process is Plasma Electrolytic Oxidation (PEO) which works in a similar way to anodization, albeit with significantly higher electrical current in the PEO process. The part is immersed in an electrolyte bath and a high voltage is passed through this electrolyte at a constant current. At this high voltage, the plasma is being generated on the surface of the part leading to micro arc oxidation. The result is a thin oxide layer on the surface that protects the part from corrosion.
‘Anodization is typically applied to aluminium components and uses acidic electrolytes to form a corrosion resistant, hardened surface layer,’ continues Laghaei. ‘Unfortunately, these electrolytes are not environmentally friendly and struggle to properly coat sharp edges and corners, which is required for the delicate fins within a heat exchanger.’
Anodization is unsuitable for a range of aluminium alloys, including the high-silicon alloys commonly used in AM, and can also suffer defects such as cracking on certain geometries such as sharp edges. PEO does not have these drawbacks as it can coat significantly more alloy types with robust, highly dense surfaces, regardless of geometric features.
How does Plasma Electrolytic Nitriding (PEN) work?
To help solve the lack of corrosion protection for aluminium parts, Conflux is collaborating with Deakin University to develop a new type of PEO process, called Plasma Electrolytic Nitriding (PEN). PEN, also known as plasma nitriding, is a surface modification technique used on various metals, including steel, to enhance surface hardness and wear resistance. It involves introducing nitrogen ions into the metal surface using a plasma in a nitrogen-containing environment (i.e., electrolyte).
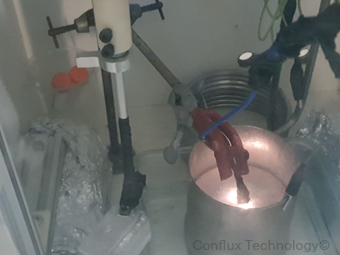
‘Our initial research shows that we can coat parts within a couple of minutes, compared to 30 minutes with traditional anodization techniques. This faster process drastically reduces the amount of energy and chemicals needed, resulting in 3D printed parts with higher performance, at much cheaper costs,’ continues Fordyce. ‘We have advanced the PEO process further by developing new coating electrolytes which contain nitrogen, the basis for our Plasma Electrolytic Nitriding (PEN) technology. PEN has proven to provide even greater corrosion protection than PEO. We started developing this for aluminium heat exchangers, but we are now realising that it can be used on any aluminium alloys and so has the potential to be used in a wide variety of applications.’
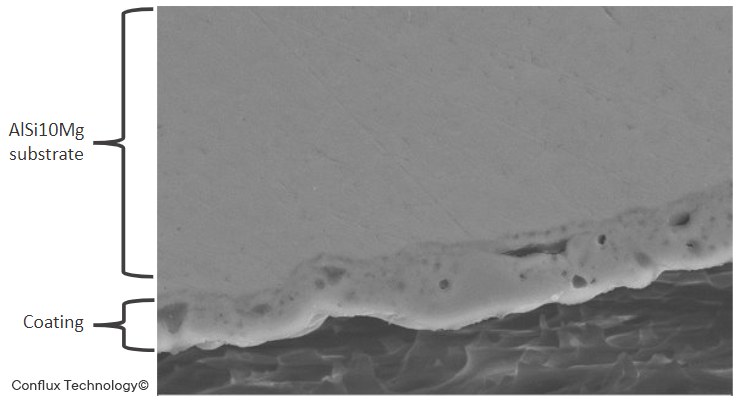
The other benefit to this innovative process is that parameters such as the time, current and voltage can be varied to tune the thickness of the coating. This ensures that for complex geometries such as micro channels and fins created through additive manufacturing, the coating is made thin enough (2 to 5µm thick, depending on parameters) to avoid impeding fluid flow. The process can also be controlled to coat specific areas of a component, leaving other areas uncoated.
Conclusion
Today’s additive manufacturing technology is allowing more complex geometries to be printed than ever before. Yet, only when the most suitable powders are selected for the right application can these parts truly achieve high performance. Innovative processes such as the PEN Conflux are developing with Deakin University have the potential to further enhance heat exchanger performance. This together with optimising printing parameters can make 3D printed heat exchangers suitable for all types of applications, from rockets to racecars.
References
[1] A,S. 2023. Unleashing the Future: Exploring the 3D Printing Market Forecast for 2030 [Online]. 3D Natives.
[2] T,A. 2022. Number of 3D printing & additive manufacturing devices worldwide from 2020 to 2030, by context [Online]. Statista.
[3] 2023. Airbus Helicopters boosts competitiveness with 3D printing [Online]. Airbus.